What are hard materials and how is Synthetic Sapphire a great option for various applications?
If you want to learn more, you’re in the right place.
Overview
This paper provides an overview of hard material definition and how synthetic sapphire compares to materials in the “hard” category. Additionally, we will review the properties, benefits and types of synthetic sapphire along with various applications for its use.
Understanding hard materials
Materials adopted in various applications where hardness, incompressibility, chemical inertness, wear characteristics, and thermal stability are desired are typically designated as “hard materials”. These materials fall into the categories of hard, superhard, and ultrahard, depending on the respective hardness values.
A material can be considered “hard” when its hardness value exceeds 10 gigapascals when measured by the Vickers hardness test. Similarly, a designation of superhard applies when the material hardness value exceeds 40 gigapascals; ultrahard materials exceed 80 gigapascals. The overall hard material category is made up of incompressible solids with high electron density and strong covalent bonds.
Applications for these materials include optical components, abrasives (e.g. polishing, grinding, cutting tool materials and wear-resistant coatings), medical implants for hip-joint prosthesis, and wear resistant components in high pressure pumps and valves; lower pressure applications benefit from superior wear characteristics, thermal stability and chemical inertness.
High performance designs capitalize on the strengths of these hard materials to meet the unique demands of the respective applications. Material searches for certain applications start with determining the optimum balance of hardness and toughness. The hardness attribute relates to component life and toughness is closely associated with the resistance of impact.
Given the choice of hard materials in the context of measurable properties, what material should be selected for an application? One could assume the choice would be as simple as selecting the material with the highest values for the various material parameters presented herein. We would be naive in ignoring one of the most important considerations in engineering an appropriate solution: cost!
Evaluating the material properties of synthetic sapphire with the list of available materials shows the obvious applicability of sapphire for applications that do not need, or account for, the shortcomings in parameters such as fractural toughness. Hardness, high thermal limits, optical properties and chemical compatibility can be leveraged at cost targets that preclude the use of materials such as silicon carbide (SiC), cubic boron nitride (CBN), or diamond. As we will discuss, the manufacture of synthetic sapphire can produce materials that meet engineering requirements without exorbitant cost, and the manufacturing techniques of the raw material have reached a level of control and automation that guarantee qualities that are dependable and affordable.
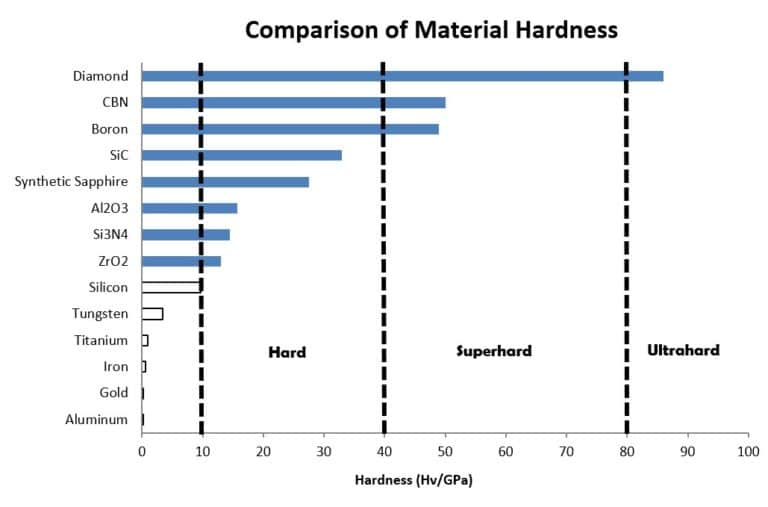
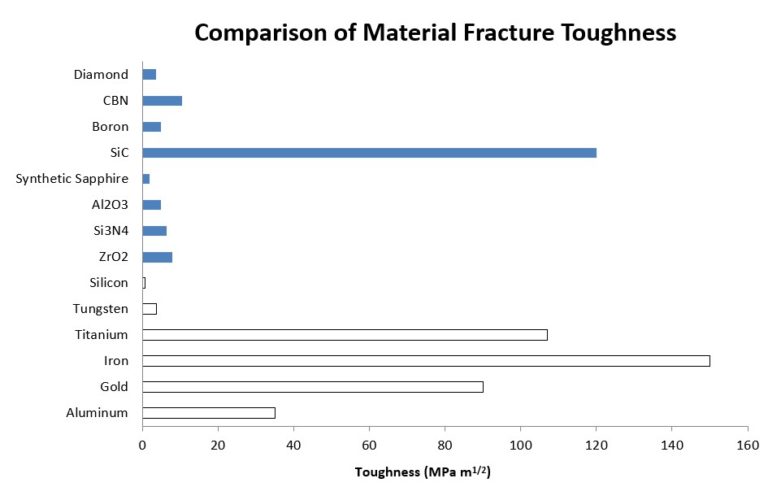
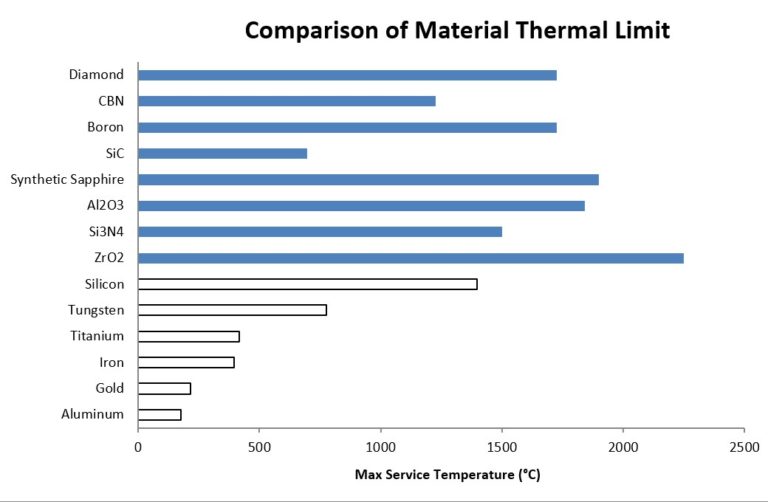
Applications of Synthetic Sapphire
Synthetic sapphire (Corundum or Al2O3) single crystal is a highly regarded material with excellent chemical stability, mechanical properties, and light transmissivity characteristics. It is often used in aggressive environments where reliability, optical transmission and hardness are required. In addition, synthetic sapphire combines zero porosity with near total resistance to acids and alkaline substances. Synthetic sapphire is insoluble in water, and only reacts with hydrofluoric acid, phosphoric acid and potassium hydroxide at temperatures above 300 degrees Celsius. Synthetic sapphire is anisotropic hexagonal crystal. Its properties depend on crystallographic direction (relative to the optical C-axis).
Applications of Synthetic Sapphire include but are not limited to the following:
- UV and IR Optics – often used as infrared filters in military and industrial applications
- Watch glasses, watch crystals, and gauges
- Silicon on sapphire wafers; Superconductor substrate
- High temperature and pressure windows, or where corrosion resistance and abrasion resistance are required
- Electrical insulators
- Thin film deposition
- Transparent electronic substrates
- Wafers
- Lenses
- High Performance Liquid Chromatography (HPLC) pump pistons and check valve seats
Synthetic sapphire, sometimes referred to as sapphire glass, is commonly used as a window material, due to its high transparency to wavelengths of light between 150 nm (UV) and 5500 nm (IR) (the visible spectrum extends from approximately 380 nm to 750 nm) and significantly improved mechanical properties when compared with other optical materials or standard glass windows. Synthetic sapphire has an Abbe number comparable to some of the highest quality glass types, which indicates the low dispersion characteristics of the material, in addition to being highly resistant to scratching and abrasion (9 on the Mohs scale of mineral hardness scale, the 3rd hardest natural substance next to moissanite and diamonds) with an extremely high melting temperature (2030 °C).
Some sapphire-glass windows are made from pure sapphire boules that have been grown in a specific crystal orientation, typically along the optical axis, the c-axis, for minimum birefringence in a given application.
The boules are sliced up into the desired window thickness and finely polished to the desired surface finish and roughness. Sapphire optical windows can be polished to a wide range of surface finishes due to its crystal structure and its hardness. The surface finishes of optical windows are normally called out by the scratch-dig specifications in accordance with the globally adopted MIL-O-13830 specification along with a roughness value for Ra.
Sapphire windows are used in both high pressure and vacuum chambers for spectroscopy, crystals in various watches, and windows in grocery store barcode scanners since the material’s exceptional hardness and toughness makes it very resistant to scratching. Thermal conductivity and wide-band transparency properties of synthetic sapphire make it an ideal choice for use as end windows on some high-powered laser tubes as it can handle very high power densities in the infra-red or UV spectrum without degrading due to heating.
As a substrate for semiconducting circuits, thin sapphire wafers are used for insulating substrates upon which depositions of silicon are used to make the integrated circuits known as silicon on sapphire or “SOS”. Other substrates can be used for the class of circuits known more generally as silicon on insulator. In addition to its excellent electrical insulating properties, sapphire has high thermal conductivity. CMOS chips on sapphire are especially useful for high-power radio frequency (RF) applications such as those found in cellular telephones, public-safety band radios, and satellite communication systems. “SOS” also allows for the monolithic integration of both digital and analog circuitries all on one IC chip, and the construction of extremely low power circuits.
Wafers of single-crystal sapphire are also used in the semiconductor industry as substrates for the growth of devices based on gallium nitride (GaN). The use of sapphire significantly reduces the cost, because it has about one-seventh the cost of germanium. Gallium nitride on sapphire is commonly used in blue light-emitting diodes (LEDs).
Sapphire has proven useful in numerous laser applications. Titanium-sapphire lasers are popular due to their relatively rare capacity to be tuned to various wavelengths in the red and near-infrared region of the electromagnetic spectrum. They can also be easily mode-locked. In these lasers, a synthetically produced sapphire crystal with chromium or titanium impurities is irradiated with intense light from a special lamp, or another laser, to create stimulated emission.
In Life Science applications, synthetic sapphire is used in Ultra High Performance Liquid Chromatography (UHPLC / UPLC) and High Performance Liquid Chromatography (HPLC) for precision high-pressure pumps used for the chromatographic separation of samples; the hardness yield superior wear characteristics between the piston and seal interface and the inertness of the material complements the wide variety of chemistries used in separation methods.
Properties and Types of Synthetic Sapphire
Synthetic Sapphire is a hard, transparent material made by crystallizing pure aluminum oxide at very high temperatures. Sapphire is a variety of the mineral corundum, also known as aluminum oxide (Al2O3). Synthetic sapphire is the hardest of all known oxide crystals with a 9 on the Mohs scale. Synthetic sapphire is second in hardness only to diamond, and retains its high strength at high temperatures.
Technical Data for Sapphire / Corundum can be found here: Data sheet
Manufacturing Processes for Synthetic Sapphire
Verneuil process
Developed in the late nineteenth century, the Verneuil process is considered the first method of producing synthetic crystals in volume. In the flame-fusion (Verneuil process), fine alumina powder (the principal mineral in corundum) is added to an oxyhydrogen flame and is directed downward against a ceramic pedestal. Adding other minerals to the aluminum oxide can create colored varieties of sapphire. For example, introducing chromium to the aluminum oxide will create synthetic ruby.
The key to the process is that the alumina powder undergoes a controlled melt as it falls through the combustion volume, forming a sintered cone on the pedestal. The tip of the cone remains in a melted state in the combustion volume, collecting additional melted powder as the process progresses.
The pedestal is lowered at the same rate that the crystal grows vertically. The alumina in the flame is slowly deposited, creating a teardrop shaped boule of sapphire material. This step is continued until the desired size is reached, the flame is shut off and the crystal cools. The Verneuil process is a flame fusion process that can create sapphires much larger than those commonly found in nature. The disadvantage of the Verneuil process is that the grown crystals have high internal strains. Boules formed with this method are limited in dimensions, e.g. less than an inch in diameter and no more than a few inches long.
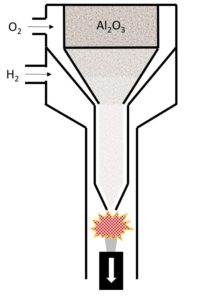
Figure 1: Verneuil Process
Figure 1 shows a “conceptual” Verneuil furnace where aluminum oxide powder is “vibrated” into a tube with a stream of oxygen gas and introduced to a combustion volume as it is mixed with hydrogen gas. The combustion volume maintains a melt volume in the forming boule as the introduced powder is melted and combined with the growth front of the boule.
Kyropoulos Process (KY)
The Kyropoulos (KY) method of bulk crystal growth is applied to grow a single sapphire crystal. The crystal growth system includes a vacuum system, heating system, cooling system, insulation system and control system. This method uses a tungsten resistance-heated element, and the heat is transferred to the thermal field through radiation. Crystallization occurs when the seed crystal contacts the melt at a temperature slightly lower than the melting temperature. Orientation of the seed crystal’s lattice structure defines the orientation of the boule that forms from the seed. The crystal gradually grows downward by water cooling from the seed holder and a programmed control of the heating power to adjust the temperature distribution of the melt. The growing boule is slowly raised, and at times rotated, to match the downward rate of growth.
The growth process is divided into seeding, shouldering, equal diameter growth, annealing and cooling. The crystals are usually boules, and the diameters are limited by the crucible inner diameters. During the growth process (see figure 2), the crystal in the crucible does not contact the crucible wall and is formed using small thermal gradients which keeps the thermal stress in the crystal lower than other production methods. At present, the Kyropoulos method is one of the effective and mature methods to grow large diameter sapphire substrate crystals and can produce high quality samples ideal for optical applications.
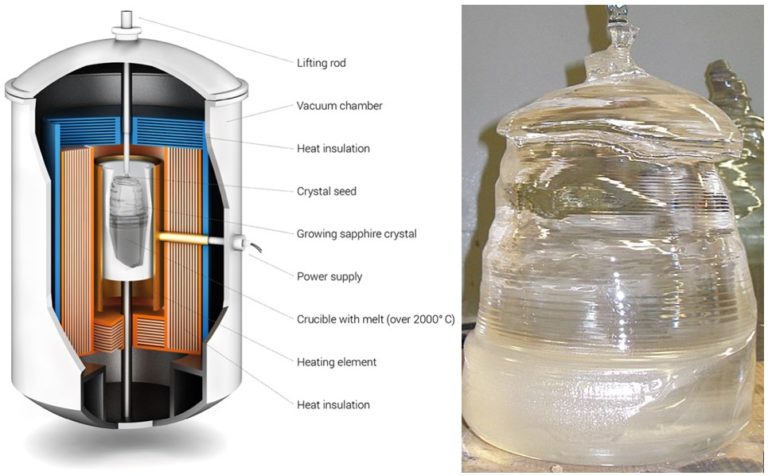
Figure 2: KY Sapphire Boules can be nearly as large as the crucible that maintains the molten raw material during formation. The KY process yields large, high optical quality materials at relatively low cost. Boules can be cleaved, cored, and/or sawed to remove volumes of materials that can be ground and polished to form sapphire components.
Czochralski Process (CZ)
The Czochralski (CZ) melt process is a method of crystal growth that melts aluminum oxide in a crucible at approximately 1500 degrees Celsius. A rod tipped with a seed crystal is inserted into the mixture and slowly rotated and pulled out, creating a column of sapphire. Although an expensive way to synthesize sapphires, it can create up to 4” of crystal per hour. The crystal is rotated and pulled at a constant rate, using melt temperature to control growth, which is different from Kyropoulos method that uses feedback to optimize pull and/or rotation. The alumina crystallizes on the end, creating long semi-regular cylindrical boules of large size up to 200 kg in mass. The main advantage of the CZ method is automatic diameter control. Many methods of manufacturing sapphire today are variations of the Czochralski process.
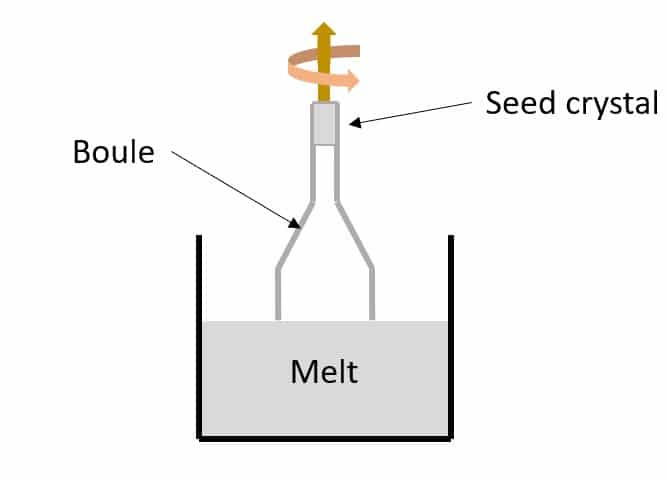
Figure 3: The CZ method looks similar to KY, but the upward direction and rotation of the forming boule are held constant as the melt temperature is varied.
Heat Exchanger Method (HEM)
The heat exchanger method (HEM) uses a high temperature heat exchanger which is introduced from the bottom of the furnace into the heat zone. There is no movement of the crucible or furnace in this method. As the heat exchange medium, helium gas circulates in the heat exchanger to take out the heat. The control of the crystal growth process is achieved by adjusting the helium flow. After partial melting of the seed, gas flow is increased to initiate crystallization of melt onto the seed. When the crystallization is complete, the furnace temperature and the helium flow are decreased, and the boule is slowly annealed in situ. During the whole growth process, the crystal is surrounded by the high-temperature melt. It is difficult to release crystalline latent heat, so the growth rate of the crystal is constrained. This process can produce nearly defect free, relatively large crystals, in a variety of lattice orientations.
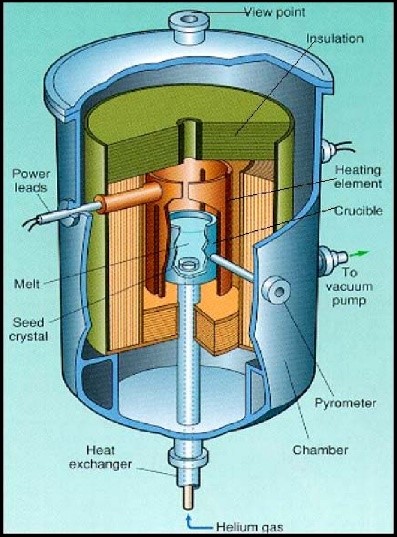
Edge-Defined Film-Fed Growth (EFG ) Method
The Edge-defined film-fed growth (EFG ) method is a technique used to grow special-shaped crystals such as ribbon, tubal and nemaline crystals. A mold with a capillary slit is put in the melt. The melt rises to the top of the mold due to the capillary force, forming a layer of thin film and spreading to the constraints of the shaper, at the same time crystallization starts from the seed that is introduced to the melt front. The advantages of EFG the fast growth rate, low cost, and multiple-piece crystals can be grown simultaneously. The main defects of sapphire crystal grown by EFG are bubbles, grain boundary effects on deviation from the shaper, dislocation and heat stress resulting from overcooling at the solid-liquid interface.
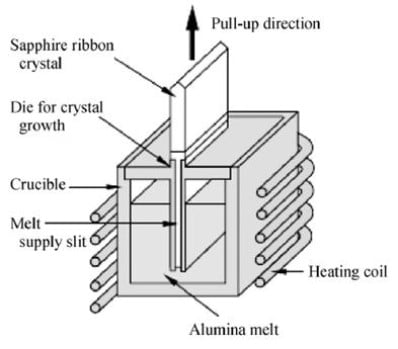
Synthetic sapphire production can leverage a variety of crystal growth technologies, each with its own advantages and disadvantages, but Kyropoulos (KY), edge-defined film-fed growth method, and heat exchanger are the most commonly used to mass produce synthetic sapphire, and Kyropoulos and heat exchanger methods are considered universal. The table below lists the advantages and disadvantages of sapphire crystal growth methods. CZ method is mainly used to grow 3″ sapphire crystals, and HEM, TGT and KY methods are mainly used to grow sapphire crystals larger than 4″. As the requirements of sapphire substrate constantly improve, the techniques to grow larger sapphire crystals are under continuous research and improvement.
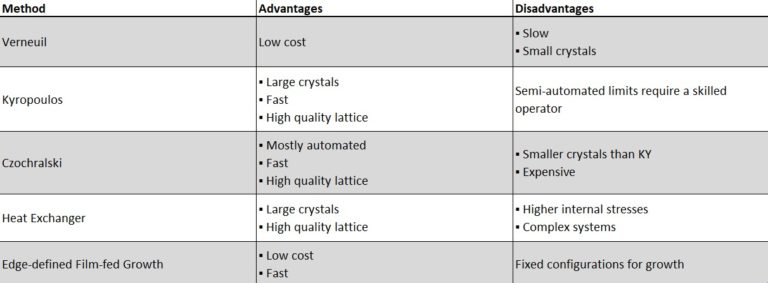
Dopants
Chemical dopants can be added to create artificial versions of the ruby, and all the other natural colors of sapphire. Artificial sapphire material is identical to natural sapphire, except it can be made without the flaws that are found in natural stones.
Conclusion / Summary
Synthetic sapphire is a robust, versatile material that can deal with harsh conditions and is suitable for many applications such as sensors, cover lenses, viewports, pressure windows and subsea imaging systems. It can withstand very high temperatures and is very resistant to chemical acids/alkaloids, high pressure and strong vacuum environments. This hard material should be at the top of the list of design engineers looking for high performance in their specific applications at reasonable cost.